The importance of making rational decisions by looking at the trends and not only the absolute values when using sensors for irrigation scheduling
The aim of this article is not to go into detail about the different sensors that can be used for irrigation scheduling, but rather to go over certain fundamental rules and basic principles that could be helpful when taking a decision based on an analysis of the measurements obtained by the sensor, as well as providing the arguments that support these deliberations.
We will mainly be discussing the aspects relating to the measurements of the soil water status, although certain principles may also be applied with regard to other types of sensors, e.g. plant water status, etc… adapting them as necessary.
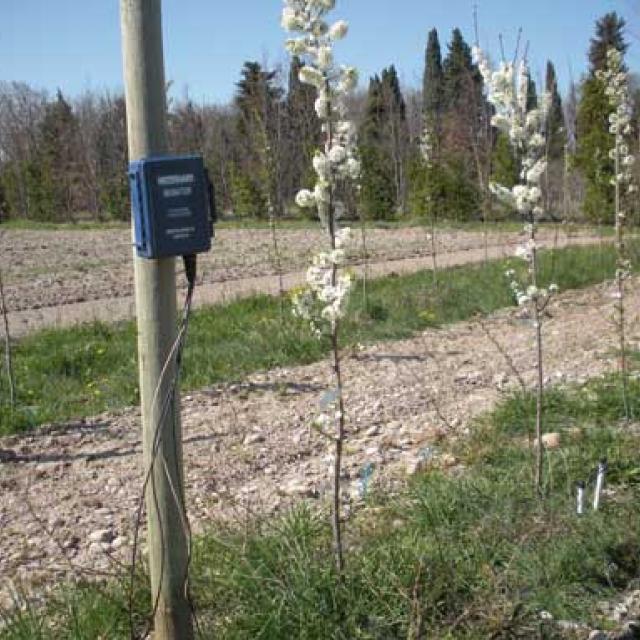
HISTORICAL REMINDER
Ever since the very first instances of irrigation used in agriculture, the form used traditionally has been the gravity-fed method. This involved the flooding of the crop area with water, without really knowing how much water was being applied to each field: water was allowed to flow into a field for a certain period of time and then the next fields were subsequently irrigated in turn.
Since mechanised irrigation came on to the scene, e.g. sprinkling and then drip irrigation, with the creation of reservoirs and closed systems, it became necessary to rationalise these amounts of water applied, notably in order to be able to forecast and plan: how much water would have to be applied in order to avoid ‘wastage’ and how to calculate the size of the installations? So, the soil was then considered as a reservoir which becomes empty when the crop consumes all the water it contained, replenished through the action of rainfall or irrigation. These processes would ‘top up the soil water reservoir’ when it reached a level beyond which the plants would have found it more difficult to absorb the water or where, consequently, there would be a risk of a decline in production (or a deterioration in the aesthetic aspect in the case of green spaces, for example).
It has also been possible to use this kind of predictive irrigation scheduling model, the ‘water balance’, to manage the irrigation in the field, in real time. However, the concepts that enable the water balance to be calculated, i.e. the soil water reservoir (‘readily available soil water reserve’, with the notion of the ‘root zone’…), ‘effective’ rainfall, ‘efficient’ irrigation, ‘real’ or ‘maximum’, or even ‘optimal’ evapotranspiration
(based on the calculation of the ‘reference’ evapotranspiration, often called ‘potential’ ET previously) are imprecise and highly variable methods, both in terms of time and area. We will not dwell on these aspects – a number of articles would be required to explain these difficulties; there is, for example, an entire FAO handbook covering the notion of effective rainfall.
Furthermore, the soil does not only function as a water reservoir: it is a zone of water movement, where the water can move downwards, e.g. to replenish the aquifers, or upwards, - known as capillary rise - or even sometimes laterally, - in the case of drip irrigation or impermeable zones, etc… However, these water movements in the ground are almost impossible to estimate under field conditions, because, if the parameters necessary for their calculation are difficult enough to evaluate on an experimental plot where the characteristics are well-defined and well-known, then it would be even more of a problem under diverse agricultural conditions.
That is why, at the end of the 70s mainly, in order to refine scheduling in real time, there was a desire to develop methods based on the use of field measurements: soil water status, plant water stress.
This involved replacing (or supplementing) these in an integrated manner, with measurements and estimates based on insufficiently reliable or assessable variables and parameters, even if these had initially been useful and necessary from a predictive viewpoint.
Nevertheless, it must always be borne in mind that a measurement or a set of measurements can only be truly useful for decision-making if the strategies used for processing them have been developed and verified. A number of teams have worked on these strategies: IRSTEA (CTGREF, CEMAGREF), SCP, ARVALIS (CTIFL)… The aim of this article is to look into some basic elements of these strategies and determine how they should be taken into account when developing the use of sensors for irrigation scheduling, particularly with the methods available today, automation, remote sharing of information, Internet…
We will mainly base this article on the use of soil water status sensors including the tensiometers.
Firstly, we will explain why we prefer these, without disregarding other types of sensors that could also be of interest (cost, ease of use, etc.). As mentioned above, certain basic principles and considerations could be adapted to very different contexts and types of measurements.
THE BENEFITS OF TENSIOMETRIC MEASUREMENTS TO IRRIGATION SCHEDULING
Tensiometry, which shows how much suction is required to withdraw water from the soil, gives us a direct indication of how much soil water is readily available to the roots, whereas the soil moisture content (percentage by volume or weight of water in the soil) does not us give any idea about this availability if the soil type is not known. For example, a sandy soil with a water content by volume of 20% can appear to be ‘waterlogged’, or at least have a water content that is very readily available to the crop, whereas a clayey soil, with the same moisture content, will almost behave like a ‘dry’ soil, the water being substantially withheld, and, therefore, not readily available to the plants…
In order to be able to use the water content concept, it would be necessary to know and understand a graph known as the soil-water characteristic curve, which compares the volumetric soil moisture content with the soil water tension or suction for each soil type, according to its texture (particle size) and structure (compacted or loose, for example). And this would apply to different layers of soil, if necessary…
It is also important to know the characteristics of the crop, particularly the positioning of the roots, for example or their absorption capacity… Another possibility to be used is that of understanding the maximum and minimum values permitted for the water content of the different layers of a soil profile and even determine how these compare with the water content values measured in real time.
This would, however, also require a preliminary study to be conducted to determine these maximum and minimum reference values.
We must emphasis, therefore, the benefit of working with tensiometric measurements rather than soil water content when we do not have sufficient information to hand about the soil and crop parameters.
Furthermore, the tensiometric measurements, taken from a number of sensors, placed, for example, at different depths, allow the user to know the direction of the soil water movement and thus avoid, or have a better control over, any possible losses caused by percolation, and/or maximise the contribution of the deep soil water reserves: benefitting the capillary rise process and saving on irrigation water by using naturally available water resources.
Finally, the tensiometric measurements are particularly useful for detecting any unusual, but not uncommon, incidents or mishaps: difference in soil types, plough pans causing waterlogging...
Numerous examples have been observed:
• plough pan waterlogging of maize in Camargue, with suffocation of the roots and poor plant growth;
• vines at the foot of a slope (Provence) with a temporary perched aquifer preventing the development of deep roots, leading to a sudden high level of water stress when this aquifer disappears in early August;
• peach trees watered by drip irrigation in the Viverais region with root suffocation in the zone covered by the drippers, and symptoms of… water stress…;
• urban spruce trees planted in pits where the managers recommend an increase in irrigation, whereas, in reality, they were ‘flooded’ and already overirrigated;
• etc...
For all these reasons (and a few others besides: cost of sensors, etc.), it would appear to us that tensiometric measurements are the type of measurement best suited to irrigation scheduling. There could, however, be reasons or occasions for using
water content measurements, particularly when
there is a good knowledge of the field references.
Anyway, the use of measurements will always be preferable to rough estimates of a water balance, which must always be treated with caution.
So, let us now discuss a few principles to be taken into account when developing strategies for using measurements for irrigation scheduling. We will focus on two main aspects:
• the benefit of using a time trend analysis of the measurements rather than referring to absolute values;
• modalities for putting these principles into practice: number of repeat measurements, representativeness, choice of location.
THE BENEFIT OF USING A TIME TREND ANALYSIS OF THE TENSIOMETRIC MEASUREMENTS FOR IRRIGATION SCHEDULING, RATHER THAN ROUGH ABSOLUTE VALUES.
This is the first principle that we wish to mention. It basically relates to initially looking at the trend of the measurements over time and not the absolute values of the measurements (without disregarding them completely, however). This principle is based on universal, or more specific, criteria
‘Universal’ criteria
• The variability of the ground conditions, often within the same field on the farm, as well as certain developed strategies (cf. bibliography) require the use of a certain number of sensors in order to be able to use these strategies. It is not, therefore, a matter of using expensive sensors applied in scientific5experiments, but rather sensors whose cost is more reasonable, although they may be less precise. Identifying the trends and not the threshold values will make it easier for the user to cope with the accuracy level of the values obtained with these less costly sensors.
• In the same vein, identifying the trends, and not concentrating on individual measurements, rather sets of measurements, will guarantee the reliability of the values observed.
• Furthermore, identifying the trends could mitigate the spatial variability of the phenomenon studied: we can confirm that the measurements in absolute terms can be extremely variable, taking into account the variability of the terrain, but the trend curve - increase or decrease of the measurements over time, change in intensity of these phenomena - are often more consistent.
• Finally, from a practical point of view, plotting the measurements on an historical trend curve allows the user to predict or forecast; the slope of the curve gives an indication of the amount of time remaining before he or she must react: rapidly, or, on the other hand, on the contrary, the farmer or manager (green spaces) may even still have time to get organised (organisation of work, watering cycles, etc.).
More specific criteria related to the observations made in the field
• The same absolute value does not have the same significance at different times of the year. Thus, it has been confirmed that in the month of August (grassland) when the soil tension value was around 20 kPa, it took 2 days for irrigation to be urgently required. At that time, the dry soil slope on the curve (increase in tension) was very steep and indicated that two days later would be too late… In September, on the other hand, the dry soil slope is not so steep, so for the same absolute value measured, we could allow more days to elapse before there would be a need to irrigate (cf figure).
• Another critical period for irrigation is the start-up at the beginning of the season. In tree-growing, i.e. cherry trees under drip irrigation, we were able to confirm that the slope of the curve for the tensiometric measurements taken in the zones watered by the drippers during the period of irrigation (see the strategies recommended for drip irrigation in the bibliography) is not steep in the long term. This means that the water being withdrawn by the roots is being replaced by the soil’s natural contribution. However, this zone, due to the fact that it has been under irrigation for a number of years, has a dense root population. At a given moment, the curve will rise sharply. That is then the time to start irrigation, but in very small doses (the measurements allow these to be adjusted), but not necessarily when the fruit producer would understandably have assumed that it is necessary to irrigate. Thus, the rooting system will continue to function properly in the zones watered by the drippers.
PRACTICAL APPLICATION OF PROCEDURES
Number of replications. Even though the strategies that allow decisions to be taken are mainly based on the measurement trend analysis, with the advantages explained above, the importance of the repeats should not be forgotten, even though the soil and crop situations are supposedly identical (at least, as far as is known….):
• with a single sensor, for a given situation, nobody is quite sure what is going to happen - particularly in the case of sensor failure - and it is not very representative
• with two sensors, if one goes in one direction and the other goes in another, no-one is quite sure what to decide
• with 3 sensors, there are two that necessarily go in the same direction, which will influence the decision; it is thus advisable to have an uneven number of sensors. For sensors that would be considered as being moderately reliable, we could instead recommend having 5 sensors, for example (instead of 3), which would allow for the extreme values to be discarded from the calculations and only take into consideration the median readings of the 3 sensors each time, possibly basing the calculations on the average of the 3 median sensors in order to maintain one single representative criteria, notwithstanding the range of conditions across the field.
Representative nature of the measurements and choosing their location. When sensors are positioned for the purpose of irrigation scheduling, the question arises of how representative they are of the zones where they are installed. However, many plots or fields, particularly - but not only - on hilly terrain, have wide disparities in soil type (high or low ground, outcrops or rocky areas, etc.), which are not immediately obvious when assessing the land at first glance. A number of different methods have been considered for obtaining a mapping system of these disparities, which have an impact on the soil-plant-water relationship.
Thus, in tree-growing, it is possible to take sample measurements of the trunks over the whole plot (work to be carried out during the off-season…); or it is even possible to take tests with a hand auger across the whole plot (painstaking…).
At the present time, the use of remote sensing is being considered in order to better understand the zones that are taken as being typical of the field or
piece of land and even be able to extrapolate the information to represent much larger areas, such as a territory or small region, for example - by grouping together zones with similar characteristics.
Finally, when positioning sensors for the purpose of irrigation scheduling, a choice will have to be made eventually: positioning the sensors in the zone with the most adverse conditions, which could lead to overirrigating of the areas with the most favourable conditions; or rather place the sensors in an area with favourable conditions, in which case the zone or zones under adverse conditions could be suffering from water stress during certain periods. This choice has to be thought out according to the farmer’s objectives (water saving, maximum/optimal yield…) and the relative size and importance of these different zones.
CONCLUSION AND PERSPECTIVES
In summary, we can say that, compared with a simple water balance approach, which is useful initially when sizing the installations, sensors are of much greater use for improving the irrigation scheduling and control in real time, whether in agriculture or the management of green spaces.
However, no sensor is completely suitable for this purpose without having in place strategies for implementing the process and interpreting the measurements taken.
We have endeavoured to mention here a few basic principles for strategies relating to the use of sensors for irrigation scheduling, as developed over the last forty years. Nowadays, the technological progress made in electronics, software, Internet and communication, particularly over the last fifteen years, has brought significant improvements and opportunities in the collection and management of data, even including automatic control systems. However, the aspects covered here in respect of using sensors and processing the information thus obtained, for the purpose of irrigation scheduling, have lost none of their importance.
The following bibliography will allow those who are interested to find further information about the examples cited above and have an in-depth knowledge of the scheduling concepts and strategies discussed above.
BIBLIOGRAPHY
BENSAOUD A., ISBÉRIE C., MARIÉ X., 2006 - Tensiométrie et fonctionnement hydrique de deux types de sols urbains reconstitués. 33es Journ. GFHN, 28-29 Nov. 2006, in: Milieux Poreux Transf. Hydr., 52, 145.
BOYER I., ISBÉRIE C., MARTINEZ A.M., BERUD M., DUFILS A., 2012 - Measuring soil water tension an soil moisture content: an experimental comparison of the new irrigation scheduling tools. Irrigaz. n° 128, 14-19.
ISBÉRIE C., 1992 - Contribution du sol à l'alimentation hydrique d'un verger de cerisiers micro-irrigué selon un pilotage tensiométrique. Thèse, Univ. Montp. II, CEMAGREF, 415 p.+ 384 p. annexes.
MÉROT A., WÉRY J., ISBÉRIE C., CHARRON F., 2007 - Response of a plurispecific permanent grassland to border irrigation regulated by tensiometer. Eur. J. Agron., 8-18.
PEYREMORTE (P.), 1984 - Pilotage des irrigations : adéquation des estimations ou des mesures ? Bull. GFHN, n° 16, 17 - 36.
RNEDHA, 1995 - La conduite de l'irrigation, de la stratégie au pilotage de l'irrigation, 124 p.
TRON G., ISBÉRIE C., CHOL P., 2013 (rééd.) - La tensiométrie pour piloter les irrigations : une utilisation raisonnée de la ressource en eau. Educagri éditions, Dijon. 250 p. (nouv. éd. revue et augmentée).